RESEARCH
Research Theme
The heart is a smart pump. In every heartbeat, cardiomyocytes generate contractile force to pump blood into circulation against a mechanical load. Like smart structures in modern engineering, the heart can sense mechanical load and adjust the contractile strength to maintain cardiac output. Our research aims to answer
two big questions: how does the heart regulate contractile strength in response to load changes in our daily activities? Why does pathological overload, such as high blood pressure, lead to heart diseases including hypertrophy, arrhythmias,
and heart failure?
Research Areas
Heart Diseases
Many forms of heart diseases increase the risk of cardiac arrhythmias. We hypothesize that a common thread linking these arrhythmias is abnormal excitation-contraction coupling and disrupted mechano-chemo-electro-transduction. Our studies will elucidate the cause of arrhythmias in seemingly distinct diseases for developing therapeutic strategies.
- High blood pressure induced heart diseases
- Muscular Dystrophy Cardiomyopathy
- Hypertrophic Cardiomyopathy (HCM)
- Dilated Cardiomyopathy (DCM)
INNOVATION
Interdisciplinary Research to Develop Innovative Technology
Our interdisciplinary team combine expertise to develop new ideas and innovative technologies. These innovations enable us to study how mechanical load such as high blood pressure impacts cardiac function and heart diseases.
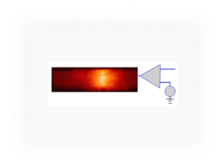
AP-clamp Sequential Dissection technology enables recording of multiple ionic currents under the action potential (AP-clamp) in a single cell by sequentially adding specific blockers to dissect out each ion channel of interest. This allows us to fingerprint the ionic currents in a single cell in order to study how the inward currents vs. the outward currents counterbalance to shape cardiac AP.
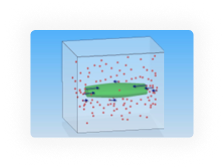
selfAP-clamp with Ca2+ cycling technology enables recording the ion current under the cell’s own steady-state action potential in physiological milieu when the Ca2+ cycling is preserved in excitation-Ca2+ signaling-contraction. This allows studying how Ca2+ transient feedback to affect ionic currents during AP.
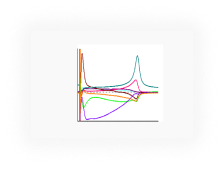
Cell-in-Gel technology embeds cells in a viscoelastic hydrogel polymer matrix to control 3-dimensional mechanical load at the single cell level by tuning the gel stiffness. This allows studying mechanical load effects and mechano-transduction mechanisms at the cellular and molecular levels.
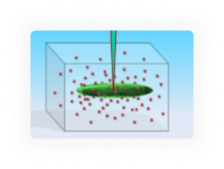
Patch-Clamp-in-Gel technology enables electrophysiology recording in mechanically loaded cells. This allows us to study how mechanical load regulates ion channels and action potential.
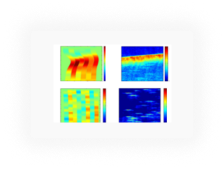
APPLICATION
High-Content High-Throughput (HC-HT) Cardiotoxicity Screening
AI Drug Discovery
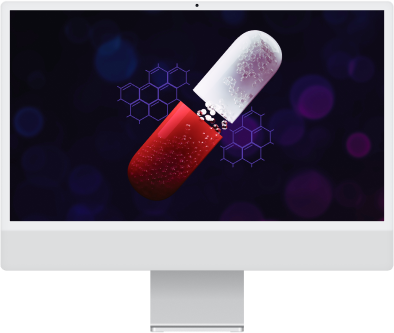
Disease-in-a-dish
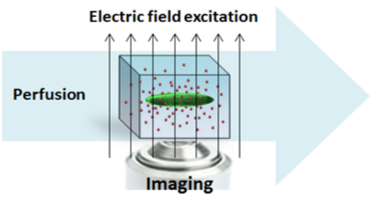
HC-HT Imaging
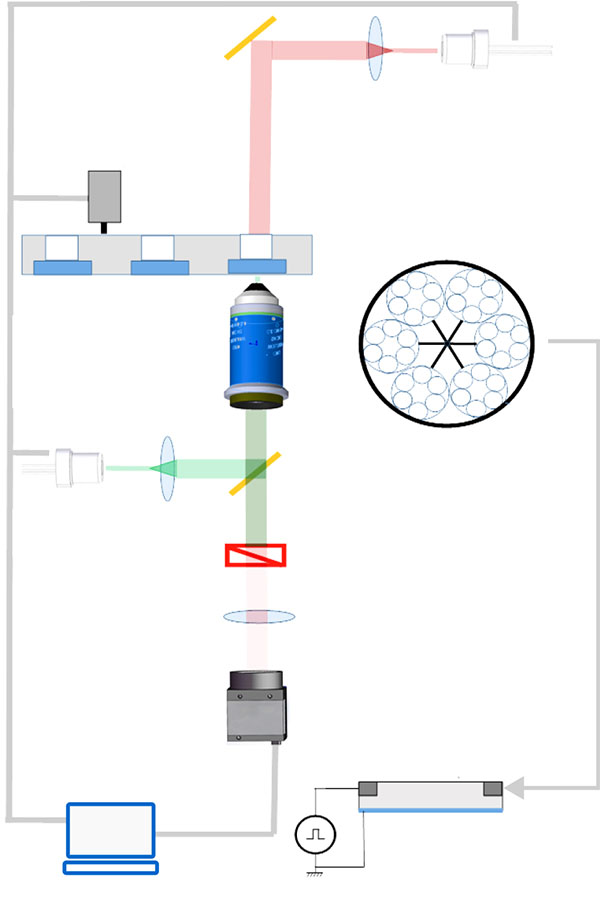